Intracontinental earthquakes, which frequently occur in densely populated regions, have catastrophic impacts on people, infrastructure, and the natural environment. This type of seismicity cannot be fully explained by classical plate tectonics theory, and its driving mechanism remains debated. Recent research from Prof. LIU Lijun’s lab at the State Kay Laboratory of Lithospheric Evolution, IGGCAS, has identified that the intense intracontinental seismicity in the Intermountain Seismic Belt (ISB) in the tectonically active Western US (WUS) is primarily caused by the interaction between mantle flow and variations in lithospheric thickness along the eastern boundary of the Basin and Range Province. This work is published in Nature Communications.
The theory of plate tectonics posits that the Earth’s lithosphere, its outermost layer, is divided into several tectonic plates that move constantly over the planet’s surface. Despite the complex three-dimensional structures of these plates, which include both the crust and the uppermost mantle, they are generally considered rigid. Consequently, lithospheric deformation is typically thought to occur mainly in narrow regions near the active plate boundaries due to the relative motion between adjacent plates, with the plate interiors being largely free from deformation. However, this classical view does not align with observed intraplate lithospheric deformation, such as intraplate earthquakes and Global Positioning System (GPS)-measured relative crustal motion, occurring hundreds to thousands of kilometers away from active plate boundaries.
The WUS exemplifies enduring intraplate deformation, featuring intense intraplate earthquakes and significant GPS-measured relative surface motion (Fig. 1). The driving mechanism behind this deformation, particularly in the north-south-trending ISB that extends from the southern Colorado Plateau to the northern Rocky Mountains, far from active plate boundaries, has been debated for decades (Fig. 1). The proposed driving forces for this deformation fall into three categories: 1) long-range plate boundary forces due to interactions between the Pacific and North American plates and the subduction of the Juan de Fuca plate, 2) lithospheric body forces arising from heterogenous lithospheric density structures and surface topography; and 3) viscous drag exerted by underlying mantle flow. Despite extensive studies aimed at understanding the causes of WUS intracontinental deformation, consensus remains elusive, largely due to inconsistent assumptions about lithospheric and convective mantle structures in previous research.
Traditional studies on lithospheric dynamics often treated the lithosphere as a two-dimensional (2D) thin shell with a presumed lithosphere-asthenosphere boundary (LAB) at a depth of 100 km. Under this thin-shell approximation, the lithosphere is assumed to have vertically uniform physical properties, and all surface topography is thought to be supported by lithospheric density anomalies above the presumed flat LAB. Additionally, the mantle flow is presumed to exert only horizontal shear along this flat LAB. These simplifications may lead to an incomplete understanding of the complex processes involved and have been challenged by recent studies.
Recent seismological and geophysical studies have revealed substantial three-dimensional (3D) variations in the physical properties of the continental lithosphere. Furthermore, many seismological studies have demonstrated significant lateral variations in the depth of the LAB on both global and regional scales. In some regions, such as the WUS, the LAB depth exhibits large lateral gradients and can reach depths of up to 250 km (Fig. 1). A continental lithosphere with laterally varying thickness may actively interact with the convecting mantle, as supported by recent geodynamic studies. This interaction generates complex surface tractions along the undulating LAB, compromising both shear and normal forces that may not have been adequately resolved in previous estimations assuming a flat LAB. Notably, the enigmatic ISB shows a strong spatial correlation with the lithospheric thickness step in the WUS (Fig. 1), strongly implying its association with localized lithosphere-asthenosphere interactions.
Given the complexity of the 3D lithospheric structure, which has often been oversimplified in previous studies, it is critical to systematically explore its links to the proposed driving forces and their associated crustal deformation. Postdoctoral researcher CAO Zebin and Prof. LIU Lijun at the State Key Laboratory of Lithospheric Evolution, IGGCAS, investigated the effects of the 3D lithospheric structure on WUS intracontinental deformation. They developed a series of data-driven, high-resolution 3D dynamic models that comprehensively simulate lithospheric and convective mantle processes within a unified physical framework. The best-fitting model not only predicted a mantle flow field consistent with recent observations of azimuthal anisotropy but also replicated the distribution of seismicity and GPS-determined surface relative motion in the WUS (Fig. 2a-c). Additionally, the model-predicted crustal stress state is consistent with recent high-quality earthquake focal mechanism solutions (Fig. 2d).
Through systematic analysis, this study discovered that the locally enhanced lithosphere-asthenosphere interaction, resulting from the thick cratonic lithosphere blocking the landward mantle flow along the lithospheric thickness step (Fig. 2a), plays a pivotal role in generating localized high crustal deformation rates and leads to the formation of ISB with intense intraplate seismicity (Fig. 2c). Furthermore, this study quantified the relative importance of proposed driving forces across the entire WUS and found that their significance varies spatially (Fig. 3). This finding emphasizes the critical need to consider a 3D heterogeneous lithosphere fully coupled with the underlying convecting mantle in accurately reproducing observed crustal deformation.
Beyond the WUS, many other regions also exhibit intense intraplate deformation and are at high risk of earthquake hazards, such as the Mediterranean, East Asia, and the Tibetan Plateau. In these regions, the lithosphere may have a complex 3D structure due to long-term thermochemical and tectonic evolution and may also actively interact with the underlying mantle flow driven by deep Tethyan slabs. Given this complexity, it is essential to consider the role of 3D lithospheric structure and its interaction with mantle flow when quantitatively studying lithospheric deformation in these regions.
This study was recently published in Nature Communications (Cao, Z., Liu, L., Western US intraplate deformation controlled by the complex lithospheric structure, Nature Communications, 2024, 15: 3917). This work was supported by the Strategy Priority Research Program (Category B) of the Chinese Academy of Sciences XDB0710000 and NSFC grant 92355302.
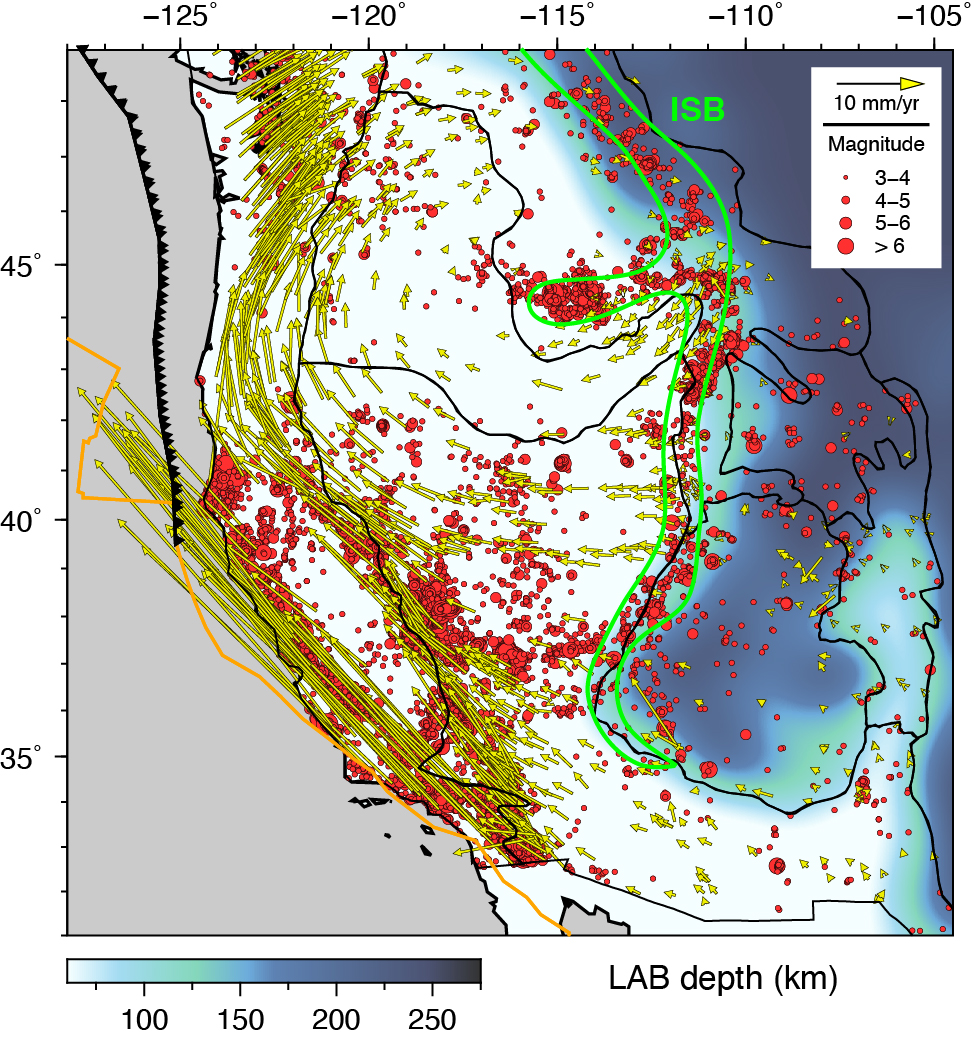
Figure 1: Seismicity and geodetically measured crustal motion overlying the LAB depth. Red dots indicate earthquake epicenters from 1980 to 2020, with the radius representing magnitudes (data from the USGS). Yellow arrows indicate crustal motion relative to the stable North American plate, as determined by GPS measurements (data from GAGE/UNAVCO). The green contour outlines the Intermountain Seismic Belt (ISB).
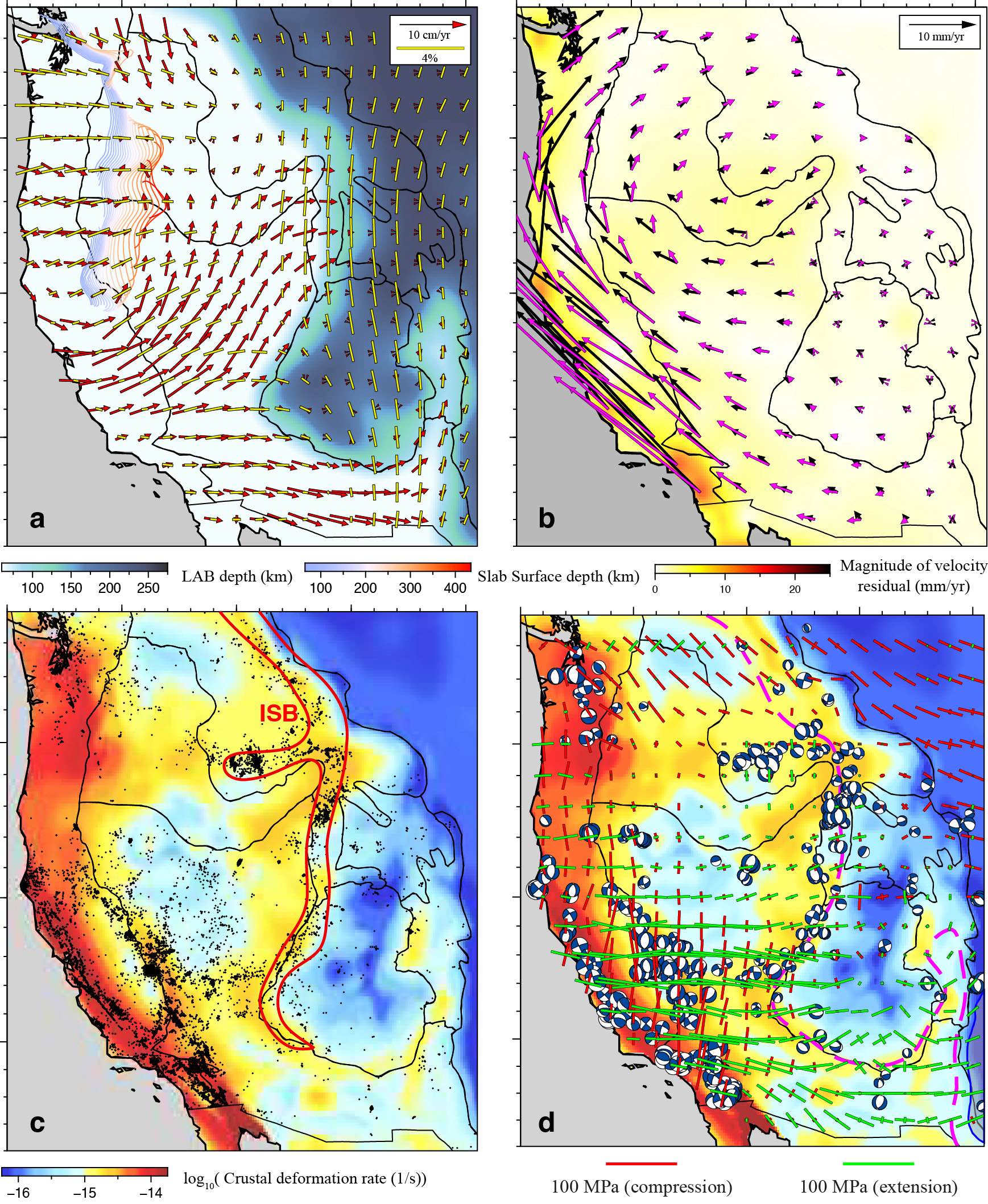
Figure 2: Comparisons between model predictions and geophysical observations in the WUS. (a) Predicted horizontal mantle flow at 150 km depth relative to the stable North American plate and observed azimuthal seismic anisotropy (yellow bars indicating the fast-propagating direction of shear waves; data from Zhu et al., 2020) at 100 km overlying the LAB depth. Colored contours indicate the upper surface of the Juan de Fuca slab at different depths. (b) Predicted (magenta arrows) and observed (black arrows) surface motion overlying the magnitude of their residuals. (c) Earthquake epicenters from 1980 to 2020 overlying the predicted crustal deformation rate. (d) Predicted crustal stress state and observed high-quality earthquake focal mechanism solutions (data from the GCMT catalog and Herrmann et al., 2011).
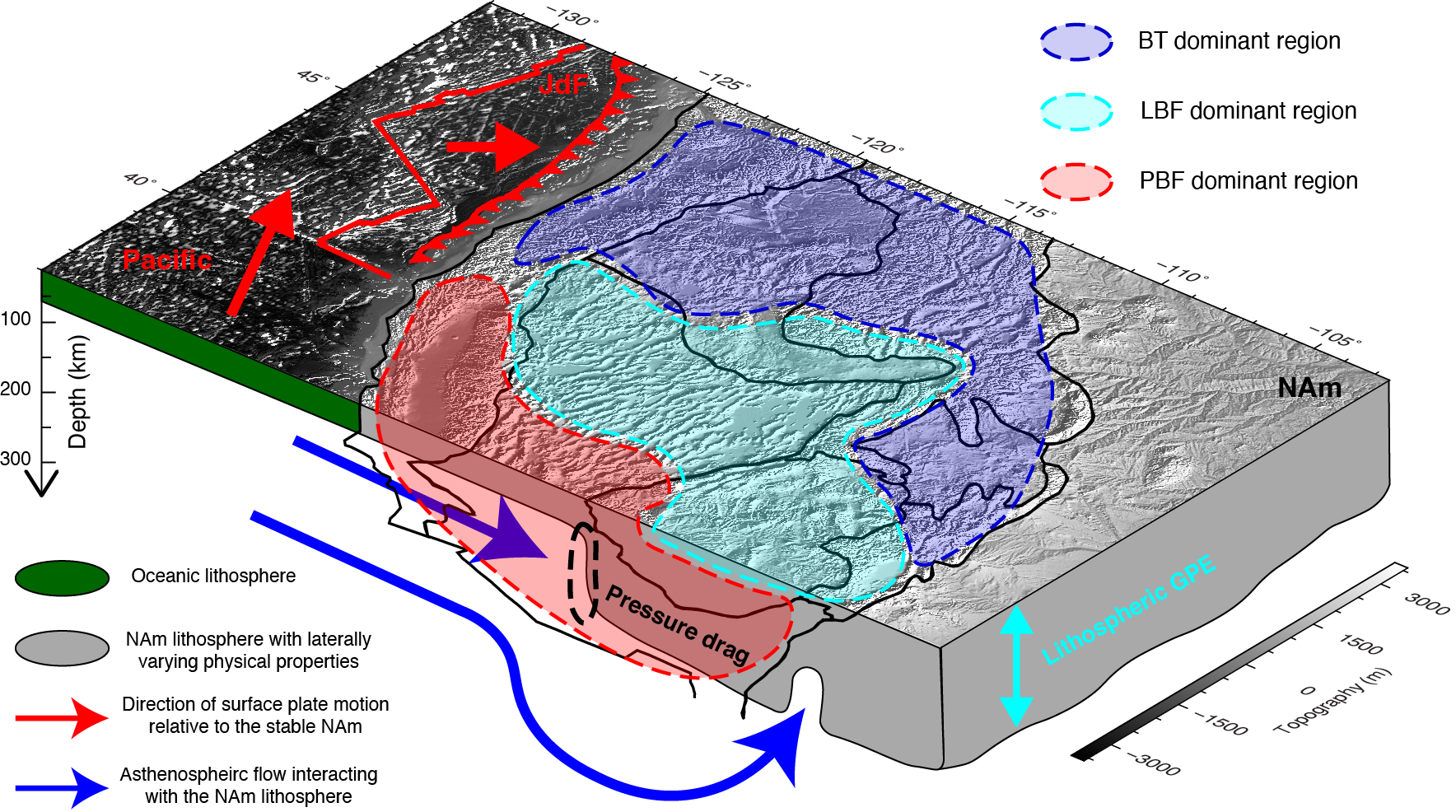
Figure 3: Schematics of the primary driving forces and their relative importance in driving WUS crustal deformation. Thin black lines indicate the major tectonic provinces on the surface. The dashed black ellipse outlines the lithospheric thickness step, where the convecting mantle applies pressure drag to the North American lithosphere. BT: basal tractions; LBF: lithospheric body forces; PBF: plate boundary forces; NAm: North American plate; JdF: Juan de Fuca plate.
Contact Information:
LIU Lijun
State Key Laboratory of Lithospheric Evolution
Institute of Geology and Geophysics
E-mail: ljliu@mail.iggcas.ac.cn